Few innovations in the history of medicine have had as profound and enduring an impact as the electrocardiogram (EKG). As both a diagnostic cornerstone and a window into the heart’s electrical language, the EKG has saved countless lives and reshaped our understanding of cardiovascular disease. Its story is one of curiosity, ingenuity, and relentless refinement, spanning centuries of discovery and innovation. This exciting history begins in the late 18th century, with a frog’s twitching leg and a spark of insight that would ultimately reveal the electrical impulses that command the heart.
In the late 1780s, Italian physician and physicist Luigi Galvani made a surprising discovery by combining his disciplines in a way nobody had done before. In his experiment, he suspended a dead frog’s hind leg on metal hooks made of iron. These hooks were attached to the frog’s sciatic nerve, and the leg was positioned so that its foot rested against a brass rail. When Galvani generated a spark by completing an electrical circuit between the metal components, the frog’s leg twitched as if it were still alive. These spontaneous muscle contractions suggested to him that some form of electrical energy was at work, even after death. Galvani argued that this was proof of an innate bioelectric force that governed life itself, challenging the prevailing view that muscles worked purely through mechanical action.
This groundbreaking work was continued by Carlo Matteucci in 1842, when he designed an experiment that allowed him to place electrodes directly on the beating hearts of frogs. To his astonishment, he found that each contraction was accompanied by an electrical current, revealing a direct link between the heart’s mechanical function and its electrical activity. This was the earliest and clearest evidence that the heart was not merely a mechanical pump, but an electrically driven organ.
Galvani and Matteucci’s pioneering experiments laid the foundation for the scientific discipline of bioelectricity, fundamentally altering how scientists perceive the forces that govern life itself. As bioelectricity emerged, it came intertwined with significant technological advancements. In 1868, Julius Bernstein developed the differential rheotome, a modified galvanometer (named in honor of Galvani) designed to detect electrical currents in the body. This device enabled Bernstein to track the time course of electrical activity in both nerve and muscle tissue, and measure conduction velocity. Using the rheotome, he provided the first accurate description of an action potential, although its underlying physiology remained unclear at the time. The differential rheotome also served as the cornerstone for future innovations in bioelectrical recording technology. Over the following decades, it was refined into the Lippmann capillary electrometer, which enhanced sensitivity and improved the precision of electrical activity recordings.
The capillary electrometer was later put to use by the charismatic Augustus Waller, a physiologist known not only for his scientific achievements but also for his passion for breeding bulldogs. Waller, who worked tirelessly in his London laboratory, followed in the footsteps of his father, the renowned physiologist who discovered Wallerian degeneration. In the lab, Waller was almost always accompanied by his beloved bulldog, Jimmy. In the 1880s, Waller recognized the potential of the Lippmann capillary electrometer, particularly its ability to measure minuscule electromotive forces. Waller adapted the device to record the electrical activity of the heart by incorporating external electrodes. These electrodes consisted of jars of saline in which the hands, feet, or even paws were immersed. When plotting time on the x-axis and voltage on the y-axis, Waller’s recordings revealed two distinct deflections—patterns that would later be recognized as ventricular depolarization and repolarization.
Through his experiments, Waller concluded that electrical activity actually precedes each ventricular contraction. This was a pivotal discovery, establishing that the electrical current was not merely associated with the heartbeat, but was in fact its direct cause. Waller’s contribution, however, was not solely in discovering this newfound understanding of bioelectricity, but also the inspiration he sparked in others through public exhibitions of his work. He was a showman at heart, and he frequently performed live demonstrations for the scientific community, showcasing his equipment and results. The star of these demonstrations was his faithful bulldog Jimmy, who would patiently stand with his paws immersed in jars of saline to display his heart’s electrical signals to captivated audiences. One of Waller’s most important demonstrations took place at St. Mary’s Hospital in 1887. In the audience that day was a young Dutch physiologist who would go on to revolutionize the field of bioelectricity—transforming it from a scientific curiosity into one of the greatest diagnostic clinical tools in all of medicine.
Although it was Augustus Waller who technically created the first EKG and demonstrated that electrical activity precedes ventricular contraction, it was Willem Einthoven who transformed the EKG into a precise, clinically valuable tool. Inspired by Waller’s demonstration at St. Mary’s Hospital, Einthoven set out to improve upon the technology. In 1897, he refined the string galvanometer, which offered far greater sensitivity and resolution than the indistinct deflections produced by Waller’s capillary electrometer. This allowed for more accurate and reliable recordings of the heart’s electrical activity.
In his early tracings, Einthoven identified four distinct deflections, which he initially labeled A, B, C, and D. However, as his work progressed through years of careful experimentation, technological refinement, and mathematical corrections for the inertia and friction inherent to the instrument, five distinct deflections consistently emerged. He ultimately labeled these points P, Q, R, S, and T. It is rumored that he chose these letters arbitrarily, but Einthoven’s decision was deliberate. Drawing from the conventions of mathematics and geometry, where such letters are commonly used to denote sequential points on a curve or graph, Einthoven applied the same methodical reasoning to his electrocardiographic tracings, treating the deflections as specific points along a continuous waveform. This nomenclature not only reflected his mathematical precision but also established a standardized language for interpreting cardiac electrical activity. His notation remains a universal standard in medicine to this day.
Einthoven published his landmark findings in a series of papers in the early 1900s, where he described not only the construction and operation of his instrument, but also detailed analyses of the electrical activity recorded from the human heart. Not only did he advance the field of bioelectricity by providing a tool crucial for discovering and understanding the cardiac conduction system, but he also fundamentally transformed cardiovascular medicine by introducing a non-invasive method to visualize the behavior of the heart, thereby enabling physicians to diagnose and treat a wide array of cardiac conditions with unprecedented clarity. His invention and scientific rigor bridged the gap between physiology and clinical practice, transforming the bioelectrical laboratory curiosities of his predecessors into a quintessential diagnostic tool. In recognition of his groundbreaking work, Einthoven was awarded the Nobel Prize in Physiology or Medicine in 1924.
Over the subsequent decades, further technological improvements, including portable machines, better amplification, and refined electrode placement, expanded the utility of the electrocardiogram. In 1954, the American Heart Association and the Cardiac Society of Great Britain and Ireland formally standardized the 12-lead EKG system, establishing uniform guidelines for lead placement, interpretation, and nomenclature, all of which are still in use today.
The EKG stands as one of the greatest technological innovations in the history of cardiology—and perhaps in all of medicine. Since its inception, it has profoundly transformed the diagnosis and management of cardiovascular diseases, becoming one of the most indispensable tools in clinical practice. The EKG has not only enabled physicians to identify and treat arrhythmias, myocardial infarctions, and a multitude of other cardiac conditions with unparalleled precision, but it has also significantly reduced mortality rates by allowing for early intervention. According to some estimates, millions of lives have been saved worldwide through timely diagnoses facilitated by the EKG, particularly in emergency settings where rapid identification of conditions like heart attacks or life-threatening arrhythmias can be the difference between life and death. Beyond acute care, the EKG has become a foundational element in preventive and investigative cardiology as well, playing a central role in exercise stress testing, perioperative risk assessment, and sophisticated electrophysiology studies that map arrhythmogenic foci with extraordinary precision. Today, the EKG remains a cornerstone of medical practice, with over 100 million tests performed annually in the United States alone, and its importance is only expected to grow as advancements in technology continue to enhance its accuracy and accessibility. Its impact on saving lives, guiding therapy, and shaping modern cardiology cannot be overstated—both as a diagnostic tool and as a testament to the power of medical innovation.
References
1. Lüderitz B. Historical perspectives of cardiac electrophysiology. Hellenic J Cardiol. 2009;50(1):3-16.
2. Cavero I, Holzgrefe H. Remembering the canonical discoverers of the core components of the mammalian cardiac conduction system: Keith and Flack, Aschoff and Tawara, His, and Purkinje. Advances in Physiology Education. 2022;46(4):549-579. doi:10.1152/advan.00072.2022
3. AlGhatrif M, Lindsay J. A brief review: history to understand fundamentals of electrocardiography. Journal of Community Hospital Internal Medicine Perspectives. 2012;2(1):14383. doi:10.3402/jchimp.v2i1.14383
4. Wilson FN, Kossmann CE, Burch GE, et al. Recommendations for standardization of electrocardiographic and vectorcardiographic leads. Circulation. 1954;10(4):564-573. doi:10.1161/01.CIR.10.4.564
5. Henderson J. Servants of medicine: augustus waller—father and son physiologists. J R Soc Med. 2005;98(4):185-186. doi:10.1177/014107680509800420
6. Sykes AH. A D Waller and the electrocardiogram, 1887. Br Med J (Clin Res Ed). 1987;294(6584):1396-1398. doi:10.1136/bmj.294.6584.1396
7. Lüderitz B. Augustus Desire Waller (1856–1922)—The First to Record ´ the Electrical Activity of the Human Heart. Journal of Interventional Cardiac Electrophysiology. 2003;9(1):59-60. doi:10.1023/A:1025328722646
8. Schuetze SM. The discovery of the action potential. Trends in Neurosciences. 1983;6:164-168. doi:10.1016/0166-2236(83)90078-4
9. Seyfarth EA. Julius Bernstein (1839–1917): pioneer neurobiologist and biophysicist. Biol Cybern. 2006;94(1):2-8. doi:10.1007/s00422-005-0031-y
10. Einthoven W. Ueber die Form des menschlichen Electrocardiogramms. Pflüger, Arch. 1895;60(3-4):101-123. doi:10.1007/BF01662582
11. Macfarlane, P.W. (2010). The Coming of Age of Electrocardiology. In: Macfarlane, P.W., van Oosterom, A., Pahlm, O., Kligfield, P., Janse, M., Camm, J. (eds) Comprehensive Electrocardiology. Springer, London. https://doi.org/10.1007/978-1-84882-046-3_1
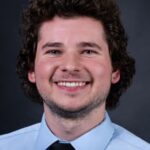
Austin Mineer
Austin Mineer is a fourth year medical student at the University of Arizona College of Medicine-Phoenix. He graduated from University of Utah, majoring in Biochemistry and minoring in Mathematics. He is applying to Internal Medicine with plans to further pursue Cardiology. Austin is an avid music collector with over 600 albums on CD and vinyl and enjoys going to concerts, hiking, learning Japanese, and playing Smash Bros in his free time. He has addictions to matcha and Mexican food. Please feel free to reach out at austinmineer@arizona.edu.